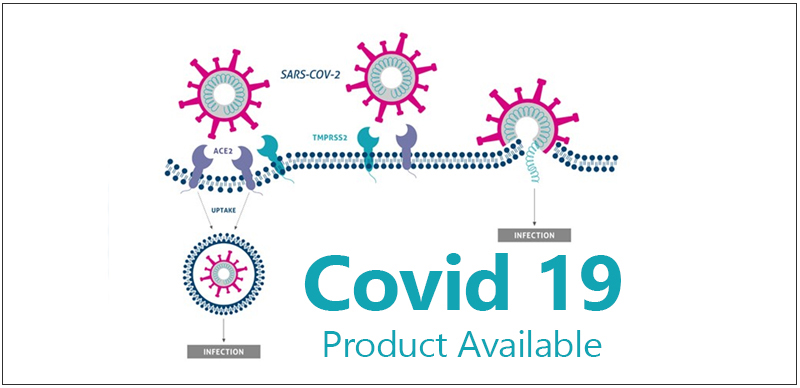
Search Products from 10 Million+
Range of ELISA Kits, Antibodies, Biochemicals, Recombinant Proteins & Assay Kits
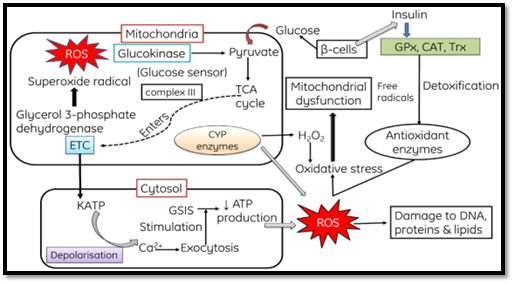
Understanding Oxidative Stress: Mechanisms and Implications
Oxidative stress is
characterized by an imbalance between the production of reactive species and
the protective capacity of antioxidants. It represents a disruption in the
pro-oxidant-antioxidant equilibrium, favoring the former and resulting in
significant damage. This phenomenon has garnered significant attention from
researchers worldwide due to its detrimental impact on the human body, being
both essential for life and implicated in cellular demise. In various
organisms, including humans, reactive oxygen species (ROS) and free radicals
are generated during metabolic and immune system processes. Molecular oxygen
(O2) possesses the ability to disassociate, leading to the formation of
unstable and highly reactive free radicals, subsequently resulting in the
generation of ROS. When the concentration of reactive oxygen species (ROS)
exceeds a certain threshold, it can have beneficial effects on various
biological functions, such as phagocytosis, apoptosis, necrosis, and pathogen
protection. In oxidation reactions, certain enzymes like peroxidases utilize
hydrogen peroxide (H2O2) as a substrate to facilitate the synthesis of complex
organic molecules in organisms.
The human body
possesses a defensive mechanism to counteract the effects of ROS, primarily
relying on antioxidants and endogenous antioxidants such as Catalase,
superoxide dismutase (SOD), thioredoxin, glutaredoxin, and glutathione.
However, when ROS concentration surpasses a critical level, it can lead to
damage to DNA, proteins, lipids, and carbohydrates, resulting in oxidative
stress. Extensive research has linked oxidative stress to the development or
exacerbation of various human diseases, including ulcerative colitis, nonulcer
dyspepsia, Parkinson's disease, Alzheimer's disease, atherosclerosis, major
depression, alcohol-induced liver disease, cancer, diabetic nephropathy,
end-stage renal disease, cardiovascular disease, mild cognitive impairment, aging,
and neural disorders. The human body maintains equilibrium among DNA, proteins,
carbohydrates, and lipids. When ROS damage these essential biomolecules, it
disrupts the metabolic state and growth and development of cells, leading to
serious diseases collectively referred to as oxidative stress. For instance,
ROS generation causes damage to nitrogenous bases and strand breaks in DNA,
with various radicals like superoxide radical (O2), hydroxyl radical (.OH), and
hydrogen peroxide (H2O2) being implicated in such damage. Among the radicals
generated in our body, hydrogen peroxide is particularly noteworthy due to its
ability to readily permeate membranes, longer longevity (approximately 1
minute), and lack of compartmentalization within the cell. Hydrogen peroxide,
produced during oxidative stress, stands out as one of the most reactive ROS,
leading to damage in proteins, nucleic acids, carbohydrates, and lipids,
ultimately culminating in oxidative stress.
Free Radicals and Reactive Oxygen Species
Free radicals and
reactive oxygen species (ROS) can be described as atoms or molecules with
independent existence that possess one or more unpaired electrons in their
outer valence shell. Reactive oxygen species specifically refer to free
radicals that contain oxygen atoms and are commonly present in biological
systems.
ROS can be
categorized into two main types: radicals, which include Superoxide (O2.-),
Hydroxyl (.OH), Peroxyl (RO2.), Alkoxyl (RO.), and Hydroperoxyl (HO2.), and
non-radicals, which encompass Hydrogen peroxide (H2O2), Hypochlorous acid
(HOCl-), Ozone (O3), Singlet oxygen (1O2), and Peroxynitrite (ONOO-).
There are various types of reactive oxygen
species (ROS) that can be distinguished based on their chemical structure and
reactivity. Some of the main types of ROS include:
Superoxide (O2.-):
Superoxide, although
not highly reactive itself, exhibits the property of a reducing agent by
facilitating the conversion of ferric (Fe+++) iron to its ferrous (Fe++) form.
Due to its inability to penetrate lipid membranes, superoxide remains localized
to the sites of its production. Notably, superoxide is spontaneously generated,
particularly in the electron-rich aerobic environment of the inner
mitochondrial membrane during respiratory chain activity. The endogenous
formation of superoxide and hydrogen peroxide is facilitated by flavoenzymes,
with Xanthine oxidase being a notable example, commonly activated during
ischemia-reperfusion processes.
Cu+2 / Fe+3 + O2
→Cu+ / Fe+2 + O2
Hydroxyl (.OH):
The hydroxyl radical exhibits higher reactivity compared to other ROS,
making it particularly damaging to essential biomolecules such as DNA,
proteins, carbohydrates, and lipids. The hydroxyl radical is formed through the
Fenton reaction, wherein hydrogen peroxide (H2O2) reacts with proteins and
other biomolecules containing transition metals (Fe+2 or Cu+). This reaction
can lead to severe oxidative damage within the cellular environment.
H2O2 + Cu+ /Fe+2 →OH-
+ .OH + Cu+2/Fe+3
Hydrogen peroxide
(H2O2):
Hydrogen peroxide (H2O2) is a pale-blue colored, covalent liquid that
readily dissolves in water. It exhibits mild oxidizing and reducing properties,
and while it can react with proteins and other molecules containing transition
metals, it does not readily oxidize most biomolecules. In the human body, H2O2 serves as an essential defense mechanism against
pathogens, playing a crucial role in activating and regulating the immune
system. Neutrophils, a type of leukocyte, produce hydrogen peroxide as a
primary line of defense against toxins, parasites, bacteria, viruses, and
yeast, thus contributing to the body's ability to combat various threats
effectively.
Hypochlorite
(HOCl):
Upon reacting with
chlorine, hydrogen peroxide (H2O2) gives rise to one of the most reactive oxygen species
(ROS), known as hypochlorite.
H + +
Cl- + H2O2 →HOCl + H2O
Exogenous sources of reactive
oxygen species (ROS) encompass a variety of production mechanisms, including:
- Radiation: Ultraviolet (UV) light,
x-rays, and gamma rays
- Chemicals that form peroxides: ozone and
singlet oxygen.
- Chemicals that promote superoxide
formation: Quinones, nitro aromatics, and bi pyrimidiulium herbicides.
- Chemicals that are metabolized to
radicals: Poly halogenated alkanes, phenols, and aminophenols.
- Chemicals that release iron: Ferritin and
other transition metals.
The generation of ROS from these exogenous sources occurs primarily
through Fenton's and Haber's reactions.
Fenton's reaction involves the reduction of molecular oxygen to produce
superoxide, which can further generate more highly reactive ROS. Superoxide
dismutates to form hydrogen peroxide: O2- + O2 + 2H → H2O2+ O2
Hydrogen peroxide can then react with transition metals such as iron
(Fe++) or copper (Cu+) to form highly reactive hydroxyl radicals: Fe2+
+ H2O2+ → Fe3+
+ OH + OH
The Haber-Weiss reaction involves the reaction of hydrogen peroxide with
oxygen to produce superoxide and hydroxide radicals: O2 + H2O2 → O2 + OH- + .OH
Halogen atoms like Cl-, Br-, and I- can also react with hydrogen
peroxide and be utilized by Myeloperoxidase to form more reactive hypochlorous
acid or hypochlorite: H2O2 + Cl- → HOCl + OH
Endogenous sources of ROS within the human body involve various enzymes such as monoamine
oxidase, lipoxygenase, cyclooxygenase, NADPH oxidase, cytochrome P450
monooxygenase, xanthine oxidoreductase, and nitric oxide synthase. These
enzymes play crucial roles in generating ROS at the subcellular level.
NADPH Oxidase /
Respiratory Burst Oxidase: The
stimulation of reactive oxygen species (ROS) production in phagocytic cells was
originally termed "the respiratory burst" due to the heightened
oxygen consumption observed in these cells. This process is facilitated by
NADPH oxidase, a multi-component, membrane-bound enzyme complex, and is
essential for the bactericidal activity of phagocytes. While various enzymes
are capable of producing ROS, NADPH oxidase holds particular significance. Its
activity is regulated through a complex system involving the G-protein Rac.
Xanthine Oxidoreductase: This enzyme catalyzes the conversion of hypoxanthine into xanthine and
further into uric acid. Xanthine Oxidoreductase (XOR) exists in two forms,
Xanthine Dehydrogenase (XD) and Xanthine Oxidase (XO). XD can be converted into
XO irreversibly through proteolysis and reversibly through the oxidation of
sulfhydryl groups. XOR generates significant amounts of H2O2
and O2- and is also involved in the transformation of nitrates into
nitrites and nitric oxide (NO). Additionally, it catalyzes the reaction between
NO and O2- to form the highly reactive peroxynitrite.
Cytochrome P450 Oxidase: This haem-containing enzyme is present in mitochondria and participates
in the metabolism of various compounds, such as cholesterol, hormones,
steroids, bile acids, arachidonic acid, eicosanoids, vitamin D3, and retinoic
acid, by facilitating intramolecular oxygen transfer. The enzyme transfers one
electron bound to oxygen while the second electron is reduced to water.
Myeloperoxidase:
Myeloperoxidase, a haem-containing enzyme found in neutrophils and eosinophils,
catalyzes the reaction between H2O2 and various
substrates to produce highly reactive hypochlorous acids. At low
concentrations, ROS, including hypochlorous acids, play beneficial roles in
processes such as phagocytosis, apoptosis, detoxification reactions, and
elimination of precancerous cells and infections. ROS are also involved in
signaling pathways that help maintain cellular homeostasis and regulate various
metabolic and cellular processes, such as proliferation, immunity, gene
expression, migration, and wound healing.
ROS Generation:
- Mitochondrial Production of ROS: ROS are generated within mitochondria through the release of
electrons from the electron transport chain, leading to the reduction of
oxygen molecules into superoxide (O2-). Superoxide is
subsequently converted into hydrogen peroxide with the assistance of
superoxide dismutase (SOD). Hydrogen peroxide can react with biomolecules
containing transition metals (Fe++, Cu+) and produce hydroxyl radicals
through the Fenton's reaction.
- Endoplasmic Reticulum: Cytochrome P450 complexes in the endoplasmic reticulum are
involved in detoxifying hydrophobic chemical compounds in the body,
leading to the formation of superoxide anions. The enzyme Cytochrome P450
reductase facilitates the conversion of these compounds into hydrophilic
forms.
- Peroxisomes: Peroxisomes contain enzymes like glycolate oxidase, urate oxidase,
fattyacyl CoA oxidase, d-amino acid oxidase, and 1-α-hydroxyacid oxidase,
which generate hydrogen peroxide. The enzyme catalase, found in
peroxisomes, is involved in various peroxidative reactions and converts
hydrogen peroxide into water and oxygen.
- ROS Generation by Lysosomes: This process leads to the reduction of oxygen and the formation of
highly reactive hydroxyl radicals (OH-).
- Other Sources: Small molecules like epinephrine, dopamine, flavins, and
hydroquinones can directly produce O2- through autooxidation.
Viral Infections and ROS: Many viral infections are associated with ROS generation, particularly
when intracellular and extracellular antioxidant levels decrease. ROS and
reactive nitrogen intermediates possess antimicrobial and antitumor activities.
For instance, viral infections like Sendai and influenza viruses induce
respiratory bursts in phagocytic cells, elevating ROS/RNS levels. HIV increases
oxidative stress by stimulating transcription factor NF-ĸB, cytokines, and
TNF-α, leading to the release of H2O2 from T-cells.
Additionally, hepatitis viruses directly affect the host genome, resulting in
ROS production and increased cell proliferation, which may ultimately lead to
cancer development.
Antioxidants
The term "antioxidant" is widely used but challenging to
precisely define. In the context of food science, antioxidants are substances
that inhibit lipid peroxidation, while in polymer science, they are employed to
regulate polymerization in the manufacture of rubber, plastic, and paint.
Essentially, antioxidants are substances present at low concentrations compared
to oxidizable substrates (found in various molecules in vivo) that
significantly delay or prevent the oxidation of those substrates.
Antioxidants can be classified into three categories:
- Primary antioxidants: Involved in
preventing the formation of oxidants.
- Secondary antioxidants: Function as
scavengers of reactive oxygen species (ROS).
- Tertiary antioxidants: Engage in
repairing oxidized molecules through dietary or consecutive antioxidants.
Antioxidants may be enzymatic or non-enzymatic in nature. Enzymatic
systems directly or indirectly aid in defending against ROS. Examples include
Superoxide dismutase (SODs), which remove superoxide by accelerating its
conversion into hydrogen peroxide. SOD enzymes contain manganese (MnSOD) in
mitochondria and copper and zinc (CuZnSOD) in the cytosol at their active
sites. Other enzymes like Catalase convert hydrogen peroxide into water and
oxygen, while glutathione peroxidase plays a crucial role in removing H2O2 from
human cells, requiring selenium for its action. Glutathione reductase is a
flavoprotein enzyme that regenerates reduced glutathione from oxidized
glutathione, and thioredoxin also contributes to antioxidant defense.
Non-enzymatic antioxidants, on the other hand, function as scavengers of
ROS and RNS. For example, Vitamin E inhibits lipid peroxidation by scavenging
peroxyl radical intermediates. Vitamin C and Vitamin A, glutathione, uric acid,
and melatonin react with ROS to form disulfide compounds, thus acting as
effective non-enzymatic antioxidants.
Cell damage resulting
from free radical-mediated reactions can be mitigated by enzymatic and
non-enzymatic defense mechanisms. The antioxidant system comprises both
endogenous antioxidants, produced within the body, and exogenous antioxidants
obtained from dietary sources.
Endogenous
antioxidants can be classified into
primary and secondary antioxidants. Primary antioxidant enzymes, including
Superoxide Dismutase (SOD), Catalase, and Glutathione Peroxidase, play a vital
role in inactivating reactive oxygen species (ROS) into intermediates. In addition
to these antioxidant enzymes, primary antioxidants also encompass water-soluble
compounds such as Ascorbate, Glutathione, and Uric Acid, as well as
lipid-soluble compounds like Tocopherols, Ubiquinols, and Carotenoids.
Secondary
antioxidant enzymes, such as Glutathione
Reductase, Glutathione-S-Transferase, Glucose-6-Phosphate Dehydrogenase, and
Ubiquinone, assist in detoxifying ROS by reducing peroxide levels and
continuously providing NADPH and Glutathione to maintain the proper functioning
of primary antioxidant enzymes. Copper, iron, manganese, zinc, and selenium
further enhance the activities of antioxidant enzymes.
Exogenous
antioxidants are primarily derived from dietary sources, including various
herbs, spices, vitamins, and vegetables, among others, that exhibit antioxidant
activities.
Oxidative stress
refers to a disturbance in the prooxidant and antioxidant balance, favoring
prooxidants and leading to serious damage to biomolecules. This term describes
the imbalance between the production of reactive species and the protective
capacity of antioxidants. Oxidative stress has garnered significant attention
from researchers worldwide due to its detrimental effects on important
biomolecules such as DNA, Proteins, Lipids, Carbohydrates, and others within
the human body.
Oxidative Stress and Its Impact on Disease
Oxidative stress
arises from an imbalance between the production of reactive oxygen species
(ROS) and the body's antioxidant defense, resulting in numerous diseases in
humans. Free radicals and other reactive species have been implicated in the
pathology of over 100 human diseases, including atherosclerosis, cancer, AIDS,
nonulcer dyspepsia, Parkinson's disease, Alzheimer's disease, major depression,
diabetic nephropathy, end-stage renal disease, and cardiovascular disease,
among others.
Various forms of
stress, such as oxidative stress, heat stress, and denaturing stresses, disrupt
the structure of proteins, carbohydrates, lipids, and DNA molecules. ROS, as a
consequence of oxidative stress, play a key role in the development of human diseases,
encompassing neurodegenerative diseases, immune disorders, arteriosclerosis,
rheumatoid arthritis, diabetes, and cancer. Reactive oxygen species produced in
the human body include superoxide, hydrogen peroxide, and hydroxyl radicals,
with the hydroxyl radical being highly reactive and particularly prone to
causing damage to biomolecules.
The process of
oxidative stress involves hydrogen peroxide reacting with transition metals
like iron and copper to generate highly reactive hydroxyl radicals, which can
initiate lipid peroxidation in cell membranes and oxidize other macromolecules.
Hemoglobin (Hb) and other heme proteins, with their higher oxidation state of
iron (Fe+4), are also susceptible to ROS-induced damage. Hemoglobin is a
fundamental molecule in living organisms, acting as a cofactor for various
proteins and enzymes involved in essential cellular processes, including gas
transport, redox reactions, and electron transport.
ROS, being highly
reactive, can damage various biomolecules such as proteins, carbohydrates,
lipids, and DNA. The substances that possess the capacity to scavenge ROS and
protect biomolecules from injury are known as antioxidants. Extensive research
has demonstrated that several enzymes, including SOD and catalase, vitamins
such as A, C, and E, and amino acids like cysteine and methionine, exhibit
antioxidant properties and play a crucial role in mitigating the detrimental
effects of oxidative stress on human health.
Trending Post:
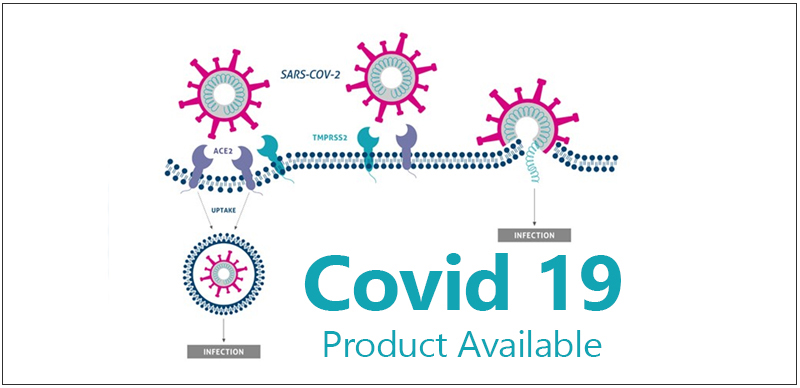
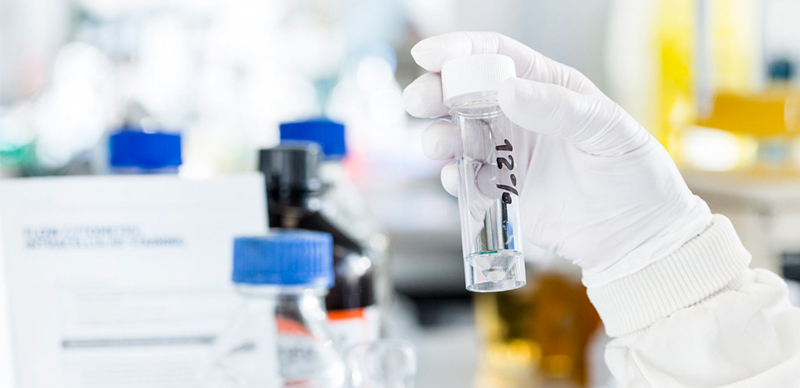
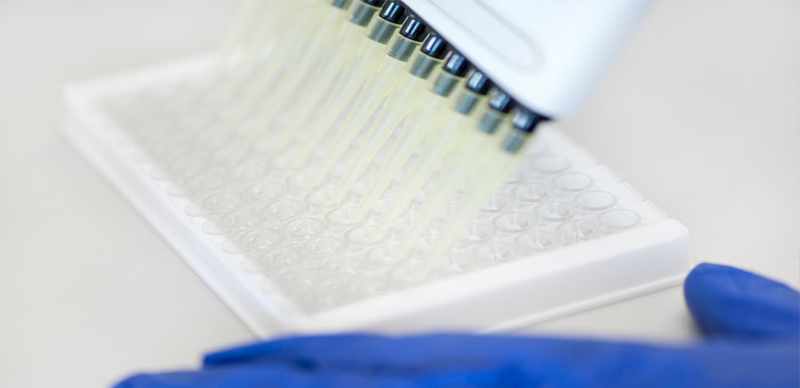
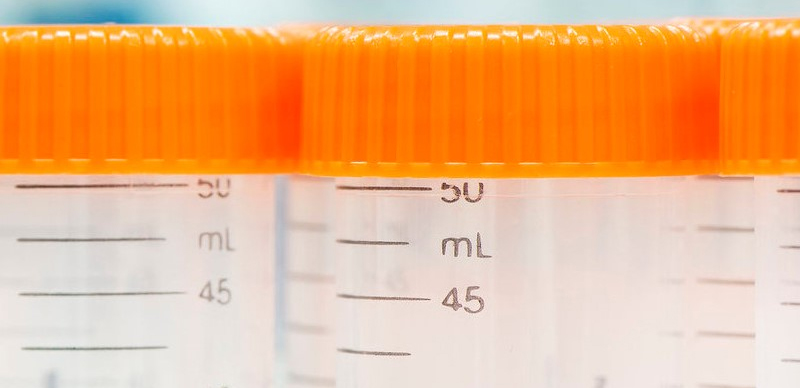
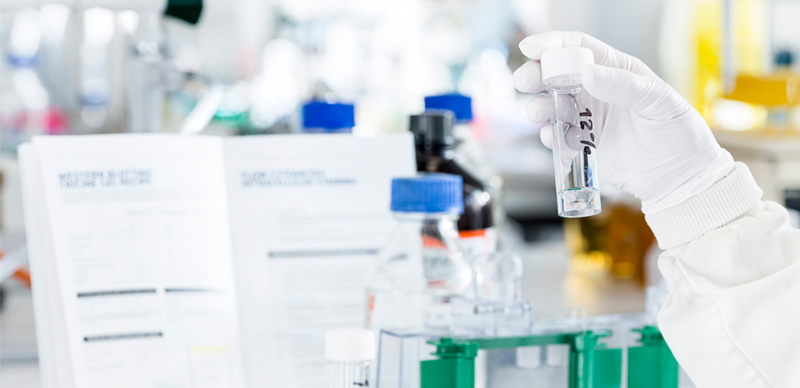
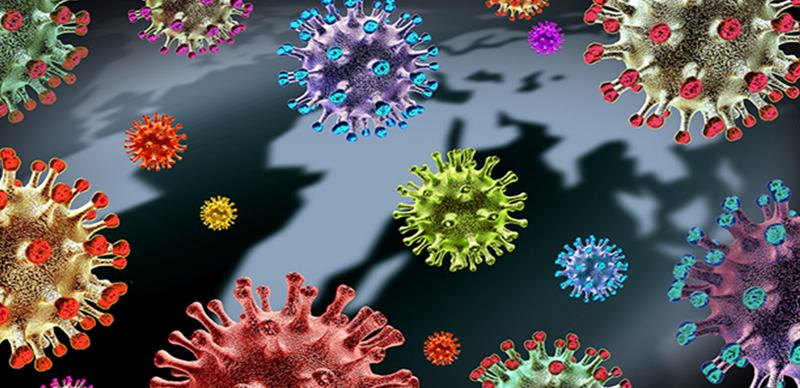
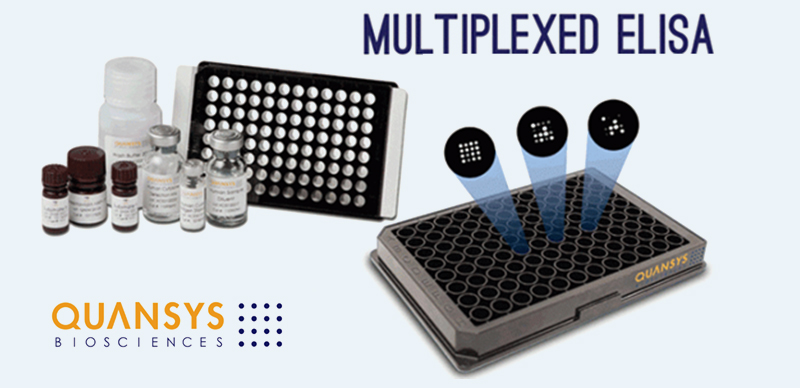
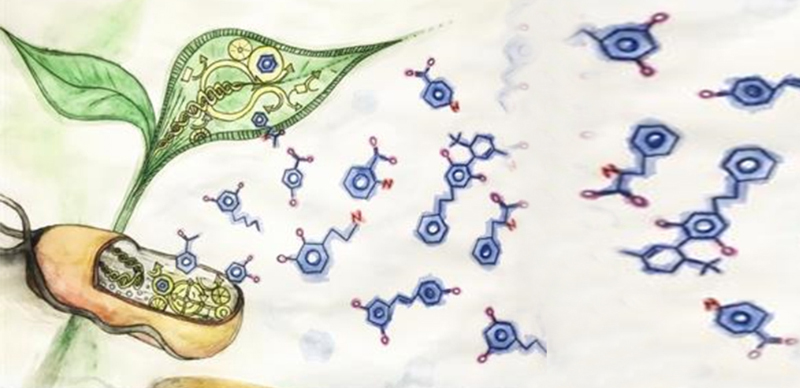
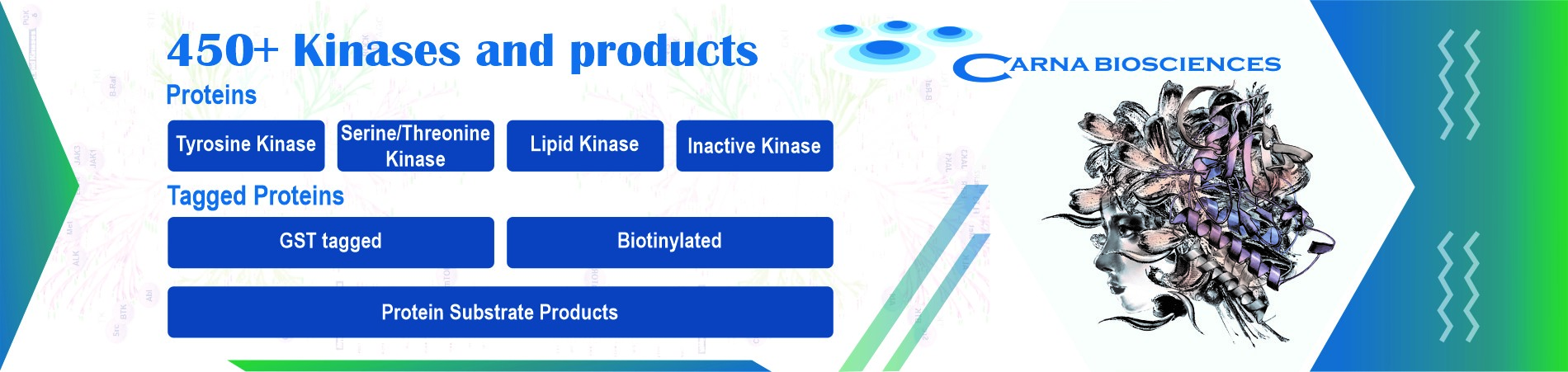
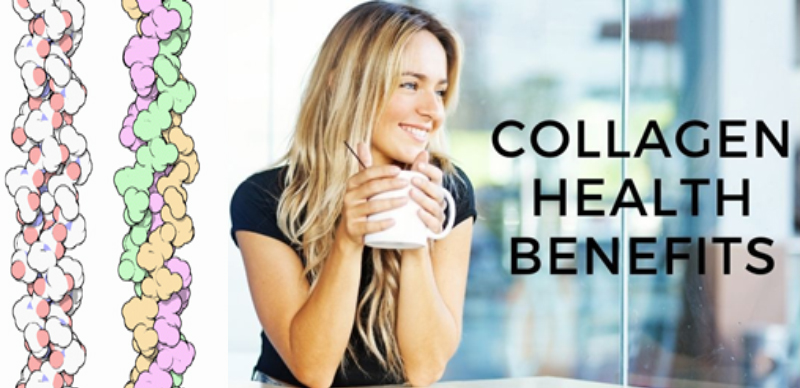
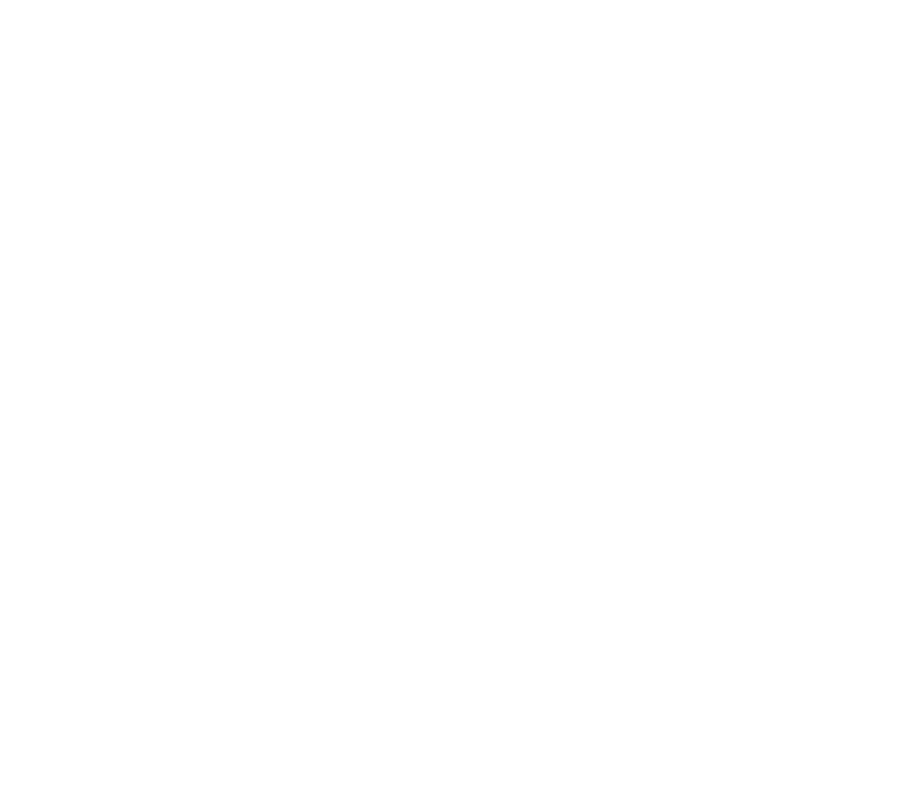
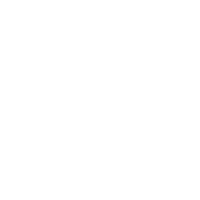
Subscribe to our newsletter
Drop your email address to get regular updates about discounts and offers